While public health workers might dive head into the main components of the opioid epidemic – which are drugs such as fentanyl, morphine, and heroin – scientists dig deeper, getting to the root of the problem which are opioid receptors, or the proteins that these drugs latch onto within the body. Where are these receptors hiding? Within the walls of cells throughout the brain and peripheral nervous system. They can be considered the “cellular gatekeepers” meaning they control the high or the euphoric feeling that comes with using these illicit drugs. They are painkillers, and they do what their name says they do, they kill the pain. Ultimately, the goal of many researchers, doctors, and professionals in this industry has been to create an opioid that gives the same relief properties without the addictive, harmful side effects. In other words, design a drug that kills pain, not people. To make this type of drug, scientists need to know the make up or the shape of its receptor. A couple of years ago, a discovery was made where for the first time the structure of this opioid receptor was observed and documented while it was bound to a drug molecule. This discovery could speed up the invention of a less addictive and therefore less deadly opioid. This idea is not as outlandish as it sounds. In the 1970’s, Scientists discovered four opioid receptors respectfully named mu, delta, kappa, and nociception. However, the mu receptor is the one that drugs such as heroin, fentanyl, morphine, and oxycodone prefer. This can be problematic due to the mu receptor enabling these drugs painkilling properties, meaning it also plays a part in their addictive side effects. Nonetheless, the kappa opioid receptor doesn’t seem to have the same problem. The kappa opioid receptor mediates pain relief but it’s side effects are different than that of the mu. The side effects from the kappa opioid receptor are non-lethal, and normally consist of hallucinations and dysphoria. Also, depending on the activating drug presenting at the kappa level, it is possible that it could activate pathways to therapy, but not those correlated to the undesirable side effects. In science, this is called “biased signaling” and it focuses on the shape or structure of the receptor. In the kappa opioid receptor, when a molecule links to it, the shape of the protein is changed – which in turn sets off a system of alarms inside the cell. So, for example, scientists who made this discovery believe, that “Pathway A” gets triggered by a certain shape that the protein has since changed in to, signaling analgesic effects, or the ones that we want to see. On the other hand, if “Pathway B” is triggered by a different shape, then side effects such as hallucinations might be generated. The issue that arises, however, is that there isn’t a specifically selective drug for either pathway. One scientist likened this comparison of designing a drug without knowing the shape of the active receptor to designing a key for a lock you can’t see. Previously, scientists have used something called X-ray crystallography which consists of cramming the protein into a crystal lattice, blasting it with a high energy X-ray beam, then assessing the proteins structure based on how the beams deflects. In 2012, this is how researchers unraveled the structure of the inactive kappa opioid receptors. However, the structure of an active kappa opioid receptor, with it being far less secure or stable meant that it was much more difficult to stabilize rendering is elusive until now. The problem was maintaining the steadiness of the kappa opioid receptor, so scientists had to get inventive. Similar to poles inside of a tent, scientists used a synthetic morphine-like molecule and a small single chain antibody to help prop up the protein in its active state. This is a colossal amount of work to get one of these proteins stabilized in their active state. Some of it is luck, even with their new “tent-pole” technique. Outcomes have ranged from 21 successful crystallization’s out of hundreds. To view the detailed illustration of this cell and a breakdown of its components, please visit: cell.com.
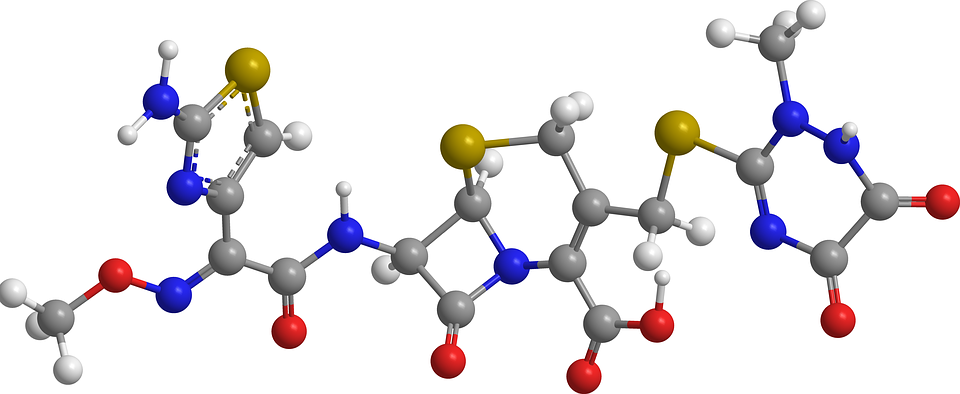
January 10, 2018